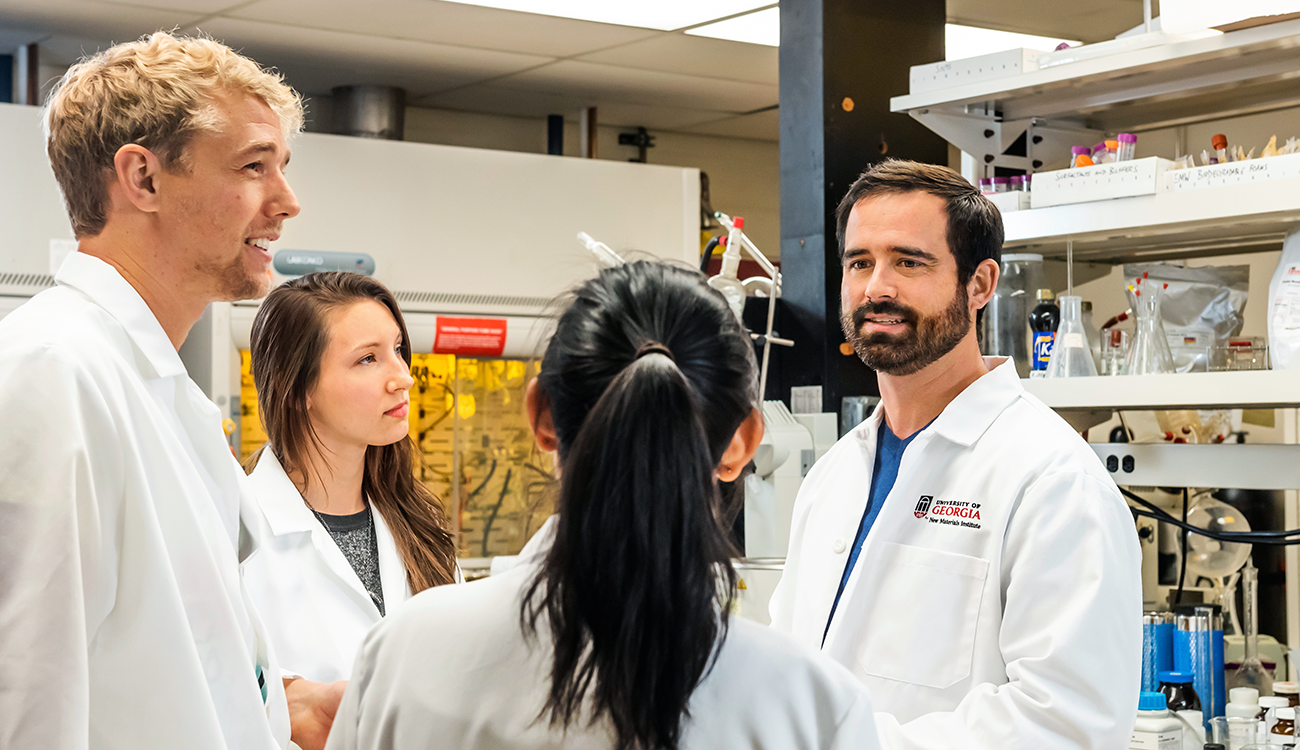
Research coming out of the New Materials Institute has helped define the significance of such a goal. A landmark study by professor Jenna Jambeck, who leads the institute’s Center for Circular Materials Management, revealed in 2015 that an estimated 8 million metric tons of plastic enter the world’s ocean every year. A follow-up study showed that 90.5% of plastic produced has never been recycled.
“In today’s economy, when we mine materials it’s a linear process—we take from the earth, we make them into something and then at their end of life, they don’t return to earth,” said Locklin, professor of engineering and chemistry. “At the New Materials Institute, we’re working as materials scientists, polymer scientists, chemists and engineers to develop next-generation solutions that won’t have the same environmental outcomes.”
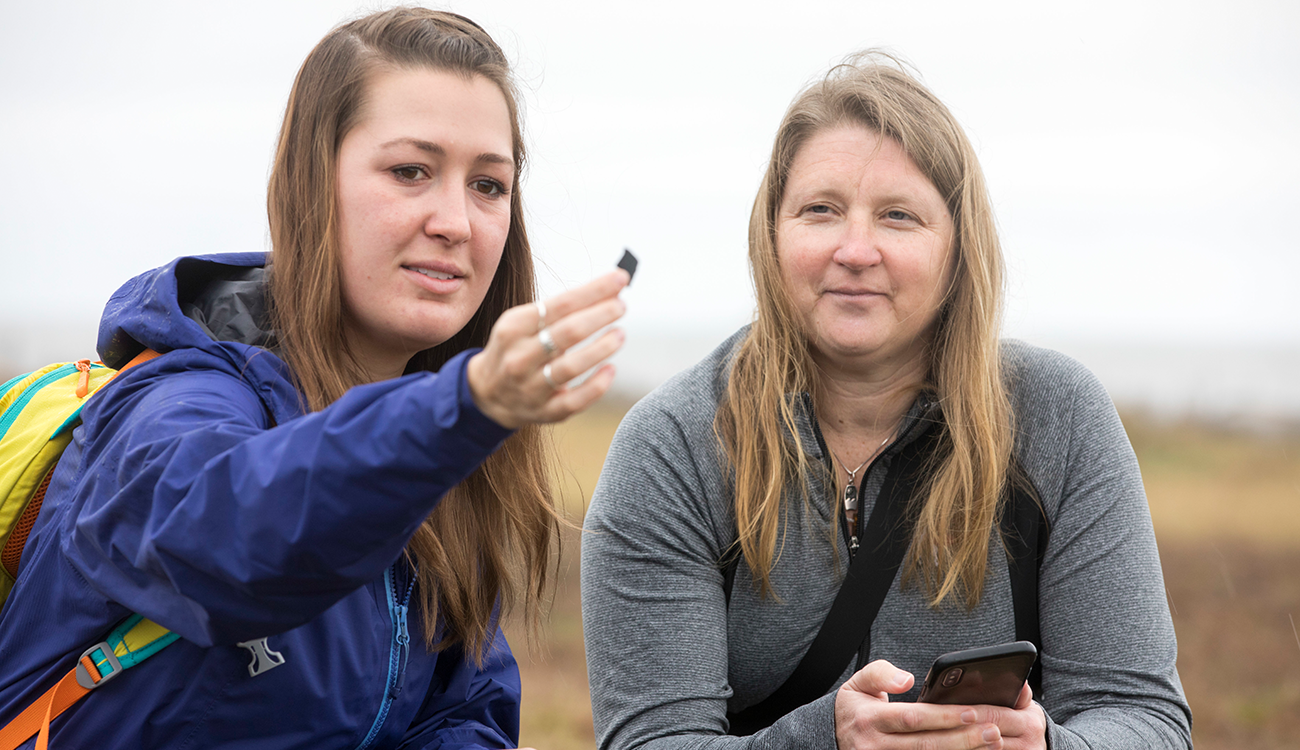
A circular mindset
One concept embraced at the New Materials Institute is designing for end-of-life, or considering in the earliest design phases what happens to a product after it’s been used. Will it break down when it reaches its final destination, whether that’s a compost site, a landfill or the ocean?
For White, the concept of designing for end-of-life is simple. He uses the analogy of a book in a library—you can store it there for hundreds of years, but if you take it outside and bury it in your backyard, it’s gone in a year.
NMI scientists are working to create something similar—materials that are designed not to be durable once they’ve outlived their usefulness.
“They’ll be shelf stable for many, many years, but the moment we put them into a receiving environment that’s microbially rich, they’ll be deconstructed into base units like carbon dioxide or methane,” he said.
“Our plastic washing up in Florida comes from the Caribbean, and what we dump on Florida makes its way to Europe. It’s all one system.”
– Evan White, NMI assistant research scientist
“The carbon cycle is the most robust recycling stream that has ever existed on Earth. It’s been going on for hundreds of millions of years. We’re trying to piggyback onto that recycling structure, but we need to understand what the microbes are capable of doing in order to access that recycling stream.”
The center’s approach also includes following the 12 principles of green engineering, developed by the American Chemical Society, which outline what makes a greener chemical process or product.
Locklin and his team aren’t just working to find biodegradable products—they’re working to find products that are compostable in everyday environments like your backyard. Biodegradable and compostable are not the same thing, although those terms often have been used interchangeably, according to White. Imagine a Venn diagram in which a large circle represents biodegradable materials. Inside that space, a much smaller circle represents materials that are compostable.
For example, PLA (polylactic acid) is the most-produced bioplastic made today. It’s typically produced from corn, and consumer perception is that PLA products can be thrown on the side of the road and will degrade. What people don’t realize, according to White, is that PLA must be collected, taken to a special facility and subjected to high temperature and chemical reaction before microbes can digest it.
“We’re trying to invent and discover new polymers and plastics that don’t have that requirement,” he said.
Locklin agrees that there’s confusion about terms like biodegradable, compostable, bio-based and bio-sourced, which leads to consumers making decisions without understanding all the consequences. One thing is clear, though—they want to understand.
“When I was a kid and went to the grocery store with my parents, we would look on the back of the package to see what was in there. What dyes did it have? Was there high-fructose corn syrup?” he said. “Today, this new generation of customer doesn’t just care what’s in the food—they also care what the package is made of and whether it’s sustainable.”
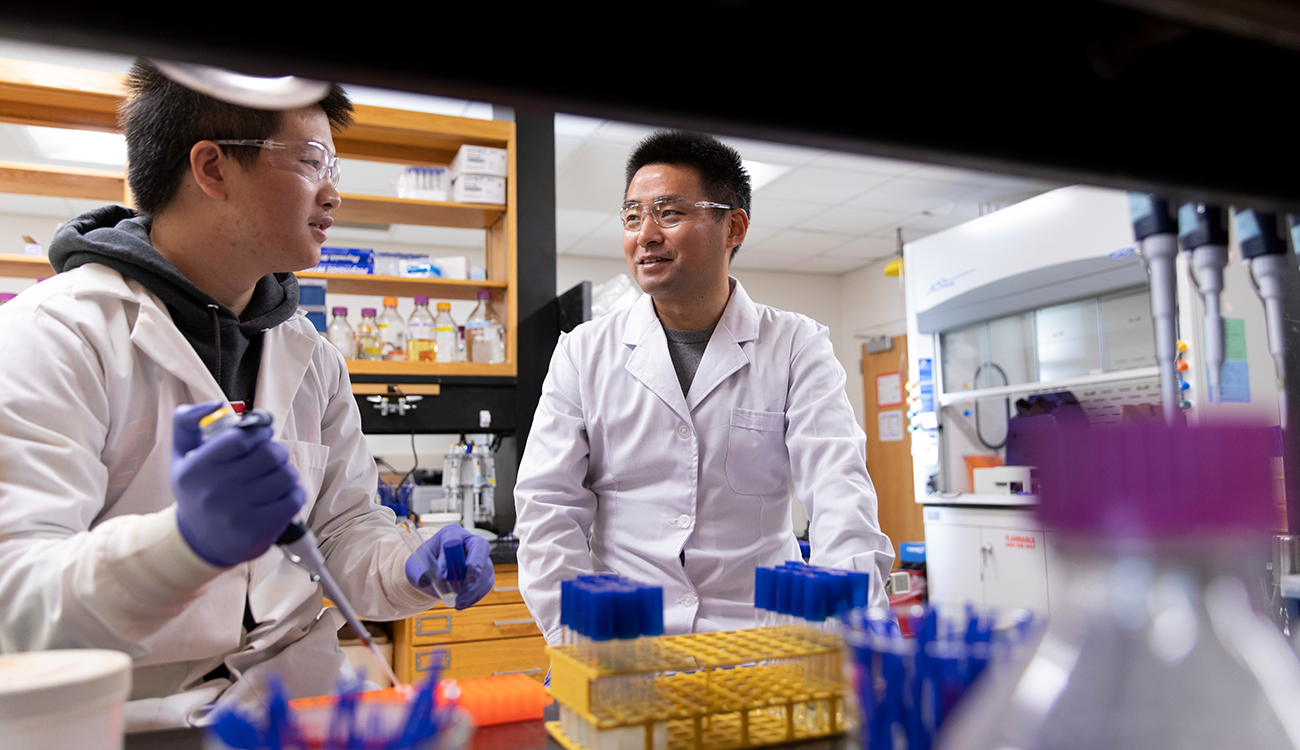
Sustainable solutions
One way the New Materials Institute is tackling sustainable packaging solutions is through a project on glutarate polyesters, led by Locklin and professor of engineering Yajun Yan.
Yan genetically modifies bacteria, using enzymatic and microbial approaches, to produce pharmaceutically important compounds, as well as fuels and renewable chemicals. He and his team took waste products from industry—for example, hemicellulose and lignocellulose from the paper industry—and used it as a food source for bacteria to convert sugars into glutarate.
“Up until Yajun’s discovery, glutaric acid or glutarate was not a bio-sourced material,” Locklin said. “You could get it from petroleum-derived products, but you couldn’t get it from a bio-based process.”
Locklin’s graduate students Apisata Holt and Yutian Ke took the glutarate and made families of polyesters that can be used in a variety of different applications, including blending them with other bioplastics to create biopolymers with superior properties. PLA, for example, is very brittle on its own, according to Locklin.
“If you’ve ever seen a compostable knife or cup, you can easily break it. It’s not very tough or resilient,” he said. “By blending those glutarate polymers with PLA, we can really improve the toughness.”
The project was funded through the Center for Bioplastics and Biocomposites, or CB2, a National Science Foundation Industry & University Cooperative Research Center that focuses on developing high-value bio-based products from agricultural and forestry feedstocks. UGA is one of four land-grant universities involved in the center, which also includes more than 40 companies.
Another project, Breeanna Urbanowicz’s exploration of xylan-based polymers, received second-year funding from CB2.
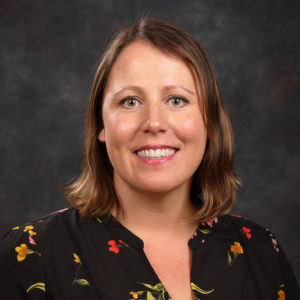
Urbanowicz, a faculty member at the Complex Carbohydrate Research Center, spent the past decade working in bio-energy based research, especially the conversion of plant biomass into biofuels. One major barrier is the plant cell wall, a carbohydrate-rich structure largely composed of cellulose, hemicellulose, pectin and lignin. That wall must be broken down into sugars like glucose and xylose in order to ferment or convert plant material into fuels or products—at least according to conventional wisdom.
“You waste a lot of energy by basically allowing plants to build these beautiful structures and then trying to break them back down,” said Urbanowicz, assistant professor of biochemistry and molecular biology. “Instead of trying to break polysaccharides down into their individual parts, we want to leave a lot of these glycosidic linkages in place and then develop chemical modification techniques to tune specific characteristics like the ability to repel water.”
Urbanowicz wants to find ways to use waste-stream components like xylan, a hemicellulose that is a major component of the plant cell wall and the second- or third-most abundant biopolymer on earth. She and Sekhar Yadavalli, senior research associate at the CCRC, are drawing on existing technologies, adapting them to modify plant-based materials and then sending them back into industry to make products. So far, they’ve successfully created coatings with different types of xylan monomers and polymers. They’re working with Locklin and White to determine if their coatings can be broken down in the average Athens composting bin.
Urbanowicz is also working with White and Locklin, plus graduate student Ethan Stinchcomb, on another CB2 project to develop faster methods for screening enzymes that can deconstruct polyurethanes, which will aid in the development of completely compostable high-barrier, multilayer packaging.
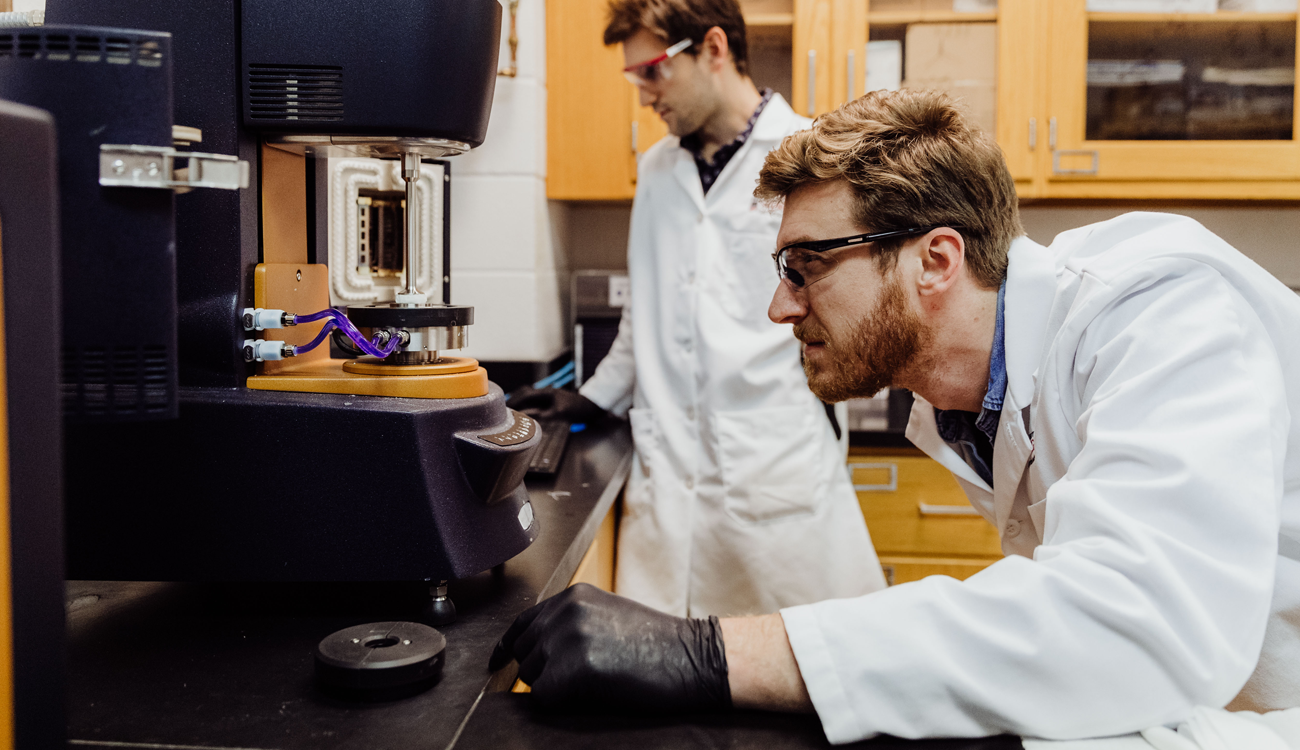
“One of the key primary products from paper and pulp industries is wood pulp, used for making tissues, paper and other hygiene products,” said Mani, professor of biochemical engineering. “Wood pulps mainly consist of micron size cellulose fibers. If you reduce fiber bundles into fibrils of nano size, you get a hydrogel-like material called nanocellulose.”
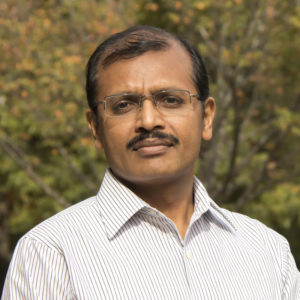
There are a number of applications for nanocellulose materials, from sustainable dye in textiles to electronics applications to biomedical applications as a lubricant. Mani focuses on sustainable food packaging.
He works with cellulose nanofibrils, or CNF, which have high tensile strength and good barrier properties that minimize the chances of gas or oxygen reaching food. But CNF is brittle, so he’s combining it with two compatible polymers: chitosan, a carbohydrate polymer primarily extracted from shellfish, and polyvinyl alcohol, a biodegradable polymer. He and his team successfully demonstrated that they could make a combination of up to 50% CNF in combination with chitosan and/or PVA.
“My research is one drop in a large ocean,” Mani said, “but we’re contributing in a way that could be extrapolated to a larger scale, including commercial manufacturing of biodegradable packaging materials.”
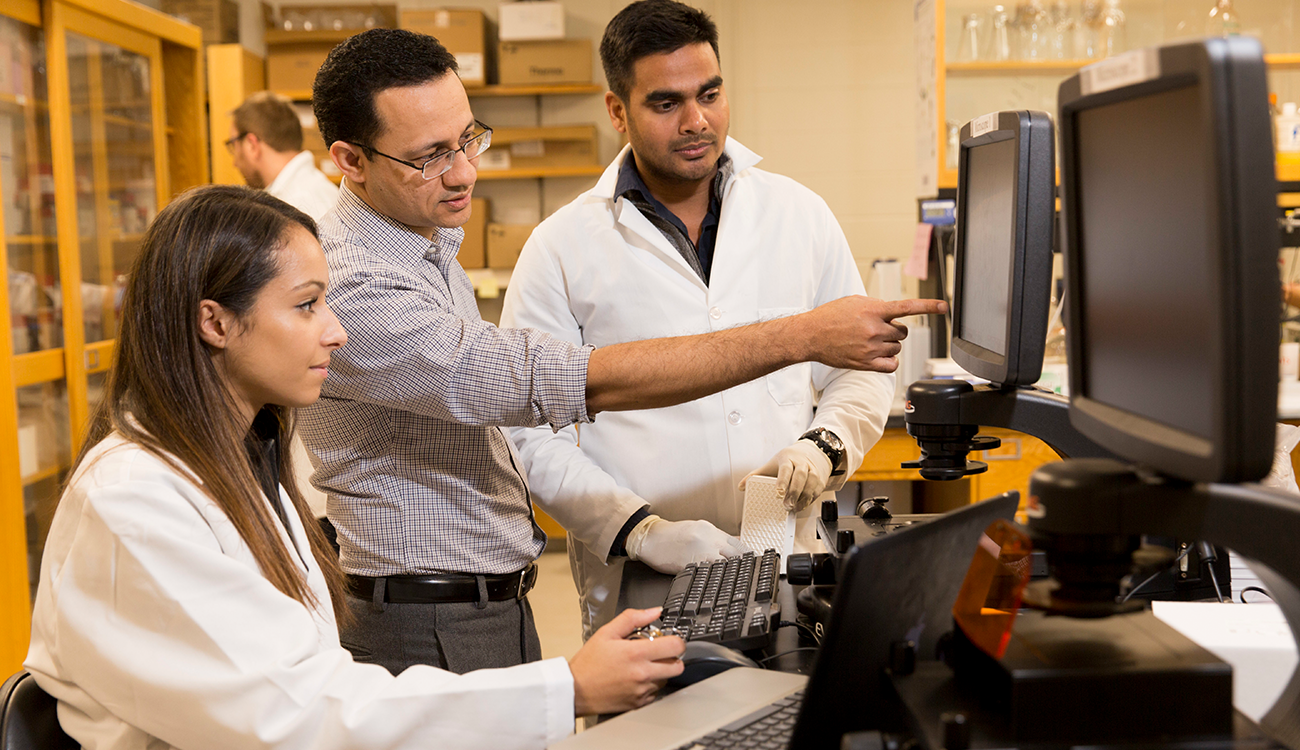
From campus to consumer
Last year, Mani got one step closer to commercial production when he and Hitesh Handa, assistant professor of engineering, received a patent on a CNF-chitosan composite coated with a biopolymer that releases nitric oxide. The biopolymer, created by Handa’s team and applied to Mani’s multilayer packaging material, demonstrated antibacterial properties.
“The next step will happen if a company wants to take it and commercialize it,” Mani said. “We’re also looking for new alternative approaches to producing it.”
For Handa, the entrepreneurial mindset is a way of life. He’s filed 12 patents, was named one of UGA’s first Innovation Fellows and founded inNOveta Biomedical. The medical device startup company is a platform to commercialize his nitric oxide-releasing polymer coating, used to prevent blood clots on medical devices like stents and catheters. The company is also developing intravascular catheters with the support of Small Business Innovation Research grants from the National Institutes of Health and Centers for Disease Control and Prevention.
“I want my work to be truly translational, going from benchtop to bedside for patients,” he said.
In the past year, Urbanowicz has started to look for outlets where her research can move into the applied arena. She went through UGA’s I-Corps program, which helps faculty and students pressure-test their entrepreneurial ideas for the market.
“Without the New Materials Institute, I would never have been able to even consider approaching this type of research,” she said. “To make an impact, we have to go bigger and get things onto the market. If we want to make bioplastics, we have to have technology that can be licensed to companies, that can produce things at a scale that makes them useful.”
One industrial partnership that’s primed to bear fruit is NMI’s work with RWDC, a company co-founded by UGA alumnus and entrepreneur Daniel Carraway to commercialize plant-based, microbially degradable polymers. They’re researching ways to create different types of polymers through fermentation, and Locklin is developing resin recipes to make single-use items and coatings—replacing the polyethylene coating in a coffee cup, for example, with something that is compostable or repulpable.
In May, RWDC announced that it would bring a new production plant—and 200 jobs—into the Athens community.
“We’ve been working with them on developing new technologies and also being a training resource to cultivate new talent,” Locklin said. “It’s a strong public-private partnership that not only helps RWDC and Athens, but also helps our undergraduate and graduate students find high-paying jobs.”
When White vacationed in the Dominican Republic, he collected several kilograms of garbage while on a beach—like this city beach in the capital of Santo Domingo. (Photo by iStock/JordiStock)
One (waste) world
Five years ago, White vacationed in the Dominican Republic. He visited the country’s most pristine beach, on the south shore, and was able to collect several kilograms of garbage in less than an hour. Naturally, he brought a sample home.
“There was a lot of Portuguese on it, so it wasn’t Dominican garbage, it was Brazilian garbage. That points to the global nature of the plastic problem in our ocean,” he said. “Our plastic washing up in Florida comes from the Caribbean, and what we dump on Florida makes its way to Europe. It’s all one system.”
With that in mind, White and the New Materials Institute are working toward consumer-based solutions that can be implemented anywhere, whether it’s a country with multiple waste streams and recycling options or a country that has none.
The institute is working to become a testing center for the Biodegradable Products Institute to test materials and provide certification of compostability. They’ve coined the term “bioseniatic” to describe a new generation of materials and products that may come from nature or be synthetic but are biologically degraded and nontoxic, making them safe for people, animals and the planet. And they’ve established the BioseniaticSM Laboratory to authenticate materials that meet their bioseniaticTM criteria.
“Once we become a certified lab, we’ll be able to accomplish all the testing necessary for that certification—respirometry, disintegration, heavy metal analysis and germination,” White said. “We also want to prove that the products are not toxic to germination, so we’ll grow plants in the residues and look down on the micro scale to see what’s going on after degradation—essentially looking a little beyond end-of-life.”
Urbanowicz thinks the demand for such solutions will continue to grow.
“When you walk down the street and see garbage or look on the internet and see sea turtles with soda rings around their neck, it becomes a societal issue that people really want to see solved,” she said.
“Companies don’t want their single-use packaging photographed and posted all over social media. People are starting to feel more and more responsible for their end-of-life uses.”
The New Materials Institute can play a leading role in helping consumers make choices about how they want to use their purchasing power, according to Locklin.
“The main reason we got into this field is really to help educate the consumer, the average Georgian who’s making the decisions,” he said. “We want them to understand the differences between the materials so they can make their own decision about whether it’s something of value that they want to support.”